Fusion power: Still a long way to go
A recent breakthrough in fusion research garnered headlines around the world. Sagarika Koppera asks Cambridge engineers what challenges may still lie ahead – and why we shouldn’t bank on fusion just yet
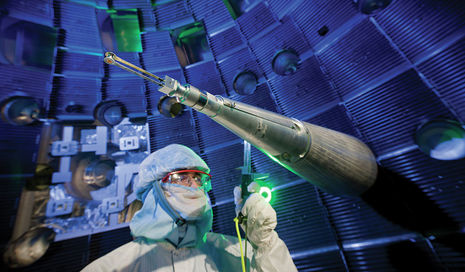
Last December, researchers at the National Ignition Facility (NIF), part of the Lawrence Livermore National Laboratory in California, made history: they reached ‘ignition’. Ignition occurs when a fusion reaction generates more energy than it consumes and becomes self-sustaining.
The facility delivered 2.05 megajoules of energy to a capsule containing the hydrogen isotopes deuterium (2H) and tritium (3H) with a laser, inducing fusion. The reaction released 3.15 megajoules (MJ) of energy, more than went into the reaction. This is the first time the feat has been achieved in the lab since research on controlled fusion began in the late 1950s.
Because deuterium is found with relative abundance in the ocean, it’s considered to have an unlimited fuel supply. Another benefit is that fusion does not produce harmful atmospheric emissions like CO2. Harnessing fusion for commercial energy could therefore provide a new, clean and sustainable energy source, so naturally the publicity around this research has been excited and hopeful.
However, commercial fusion energy is still a distant reality, and the breakthrough at NIF is only one step on a long path. The reaction lasted but a fraction of a moment and while the energy that the reaction produced was higher than the energy delivered to the target, it was nowhere near enough to break even with the total energy usage of the experiment.
To learn more about the future of fusion, Varsity contacted Professor Eugene Shwageraus, Professor Geoff Parks and Dr Paul Cosgrove, researchers in nuclear engineering at the University of Cambridge. They explained that the reaction must become far more efficient still, increasing energy production (or reducing input energy) by orders of magnitude. They also highlighted the challenges that will be faced in converting the reaction’s output into useful electrical energy on a commercial scale – a process which has yet to be demonstrated.
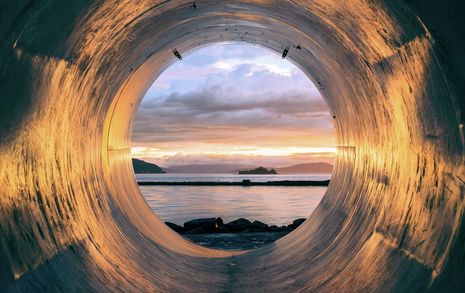
All three researchers mentioned that, despite an essentially unlimited, and therefore low cost, fuel supply, the total cost associated with fusion is not insignificant and presents another major hurdle to commercialisation. For example, neither supplies of uranium (used for fission) or of deuterium (used in fusion) present inherent constraints to these methods: indeed, Prof. Shwageraus pointed out that fuel supply made up only a fraction of the cost of existing fission reactors and the same would be seen for fusion. “Both fission and fusion have essentially unlimited fuel, and in either case, this does not imply cheap electricity.”
There are additionally many cost uncertainties associated with the design, construction and maintenance of fusion reactors and power plants. These have already plagued fusion research; ITER is the largest fusion project in construction today, at a planned cost of $22 billion. The US Department of Energy (who are contributing financially to the project), however, have projected the costs to be even higher still: a total of $65 billion, tripling the already large bill. ITER disputes this claim.
Reactor materials will also need to be constantly replaced due to bombardment with high energy neutrons, as they may become ‘activated’ under these conditions. While the neutron activation products produced in a fusion reactor are relatively short-lived, they require the same kind of disposal measures as any nuclear waste. Prof. Shwageraus explains: “although the volumes and activity of the waste would obviously be orders of magnitude smaller than in fission reactors, the requirements for regulatory oversight and radiation protection would not be possible to eliminate entirely.”
The need for robotic handling of these components will inevitably add onto costs. Taking into account the total costs of a fusion powerplant, it becomes clear it will likely be no cheaper than fission or renewables – and if it does become commercially viable, the question remains whether it would be competitive.
While the recent breakthrough was a huge milestone, the challenges associated with commercial fusion energy make its future still uncertain. Fusion energy is unlikely to become a viable solution to the global fuel crisis or to play a significant role in climate change mitigation in the immediate future. It is therefore important that any hope for commercial fusion energy should not distract from investment in currently available low-carbon forms of energy such as nuclear fission, wind, solar, hydroelectric and bioenergy, all of which are well established and contributing to the National Grid.
News / Proposed changes to Cambridge exam resits remain stricter than most
7 May 2024News / Cambridge students set up encampment calling for Israel divestment
6 May 2024News / Pro-Palestinian students disrupt Peter Thiel talk at Cambridge Union
9 May 2024Features / Cambridge punters: historians, entertainers or artistes?
7 May 2024Sport / The ‘netball girl’: myth or reality?
7 May 2024