How natural scientists joined forces to reveal how plants work
Andrea Rogolino explains how great discoveries can result from simple concepts when collaborating
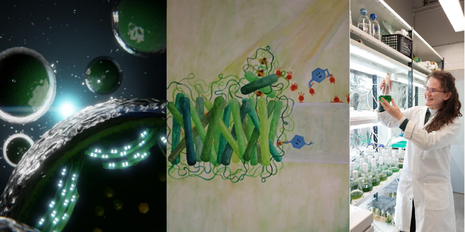
Back in my days as an undergrad, I have often come across what I later enjoyed referring to as the ‘derby mindset’ of higher education students. In football, a ‘derby’ is a match between local rivals, that is, teams belonging to the same city. Despite sharing the same home, local history and ideals, supporters of different teams engage in ferocious fights only motivated by pride: a pride whose rationale nobody understands, but just makes people feel better. Likewise, maths students despise engineers for their lack of formality, chemists accuse biologists of ignoring the laws of thermodynamics, and experimentalists mock theoreticians for being too speculative. To me, all these squabbles sounded like nonsense. Weren’t we pursuing the same goal – learning new things – just with different tools?
Eventually, you graduate, grow up and realise how often hidden pieces of knowledge lie at the boundary between different disciplines. That is what a team of researchers from the Department of Physics, Biochemistry and Chemistry demonstrated when they joined forces to peer into one of the most interdisciplinary puzzles of science: photosynthesis.
“Photosynthesis is one of the most interdisciplinary puzzles of science”
Their results are now out in Nature. In a nutshell, photosynthesis is all about using the energy of sunlight to move electrons from one side of the cell to the other. These tiny, charged particles leave water (that is oxidised to oxygen, which in turn we breathe) to finally reach carbon dioxide (that is reduced to sugars, the source of energy for plants) somewhere else far away. Unfortunately for us, this delivery occurs very quickly, within billionths of a second. Because of that, people could only understand what plants do “from a certain point on”. Now, scientists at Cambridge have managed to intercept and extract very early electrons – generated immediately after a beam of light hits the leaf – which is pretty much equivalent to catching a bullet the moment it comes out of the barrel.
To do this, the authors of the work have combined living cells and lasers to look at how fast electrons move, using a technique known as Transient Absorption Spectroscopy (TAS). Continuing with the ballistic analogy, here, the trigger is pulled with a laser pulse, and dynamic footage of the bullet is taken within tiny fractions of a second using a continuous optical beam. That sounds like a complicated experiment, so I asked Tomi Baikie, co-first author of the work and NanoFutures Fellow at the Cavendish Laboratory, Department of Physics, how difficult it was to combine biology with optics. “Surprisingly, it was much easier than expected once we optimised for the biology”, I hear his reply with some surprise, “You can shine red light on photosynthetic cells in benchtop instruments, simulating the light from the Sun. The novelty of this work was to study the cells in vivo, that is in their whole, which can give more information than simply studying isolated pieces”.
Leaves are green, which means they filter out red from the light they receive from the Sun. When a continuous optical beam (the high-speed camera of the analogy) is shone on the leaf, the intensity of the red portion of such beam passing through the leaf decreases, which can be verified with a detector. This happens precisely because electrons continuously absorb red light. As soon as a strong red laser pulse (the trigger) is shone, electrons move to a high energy state, where they do not absorb red light anymore. However, this lasts for a very short time, because immediately afterwards they start being transferred, recovering the original, red-absorbing state. In the detector of the laser beam, the signal experiences a sudden jump after the laser pulse and then springs back to the original value within a certain timeframe. The variation of this signal in time is the output of a TAS experiment. The time needed for the signal to be recovered gives information on the speed at which electrons move and their energy state frame by frame.
“Cambridge scientists managed to intercept early electrons, the equivalent of catching a bullet as soon as it leaves the barrel”
Interestingly, we can study the same dynamics by sticking cells to electrodes (like those in batteries) and capturing the electrons generated by light. “We don’t know why they do that, but certain photosynthetic organisms spit out electrons naturally”, says Robin Horton, PhD student from the Zhang Lab in Chemistry. Again, I was expecting immobilising living matter on artificial electrodes to be a tricky task, but Robin reassures me, explaining that “by using highly porous materials as supports, our creatures happily stay there”.
It became clearer and clearer to me that what made this discovery into a high‑impact journal was not the use of advanced experimental methods or theories but rather the courage to embark on a collaborative effort. Plant Scientist Laura Wey cultivated, nurtured, and engineered living cells. She then handed them over to the Cavendish Laboratory ready to be scrutinised under laser beams in a dark room. “The idea was conceptually really simple” argues Tomi, “What made all the difference was this little term here” he continues, highlighting the word “ultrafast” next to “spectroscopy” on his laptop. He explains that ultrafast (thousandths of billionths of a second timescale) optical methods are not new, but they are usually dedicated to exploring materials for devices like solar cells. “We really stumbled upon a really interesting niche”, concludes Tomi. The study also has had a lot of resonance for its potential practical implications. If we can capture electrons energised by light before they are transferred farther in cells, we can potentially “wire” photosynthesis and utilise those charges in solar cells.
However, maybe we should stop asking science for technological revolutions for once and just appreciate the beauty of discovery. When I ask Robin why we should continue studying photosynthesis, they initially remark that “plants take sunlight and turn it into energy, so if we study that, we can hack into it and maybe solve the energy crisis”, but after a short pause they add “ … probably not and we’re all gonna die of global warming regardless of the research I do. Solving the climate crisis will surely require a collective and interdisciplinary action”. Then they add, “Photosynthesis is just so clever. It has evolved for 3.4 billion years. I just like studying it because it’s really cool!”
We will always enjoy derbies, but sometimes we must accept how big an impact we can make with simple ideas by joining “rivals”. And Cambridge is an excellent place to do that.
Comment / How a culture of knowing shapes the Cambridge application process
1 March 2025News / Murder investigation launched following Chesterton Road stabbing
27 February 2025Theatre / L’incoronazione di Poppea: lessons in power and patience
1 March 2025News / Robinson swaps May Ball for a ‘mega bop’
26 February 2025News / Pro-Palestine protesters hold rally during graduation ceremonies
2 March 2025